Introduction
Psoriasis is a complex chronic inflammatory skin disease, mainly characterized by the abnormally proliferated and differentiated keratinocytes as well as the infiltrated pivotal lymphocytes including dendritic cells and T cells into dermis and epidermis [1, 2]. Patients with psoriasis have high risks of consequent complications such as gastrointestinal diseases, chronic kidney diseases, cardiometabolic diseases, and malignant carcinomas, thus making it a serious health and global issue [3–5]. It is estimated that the annual medical cost of psoriasis patients in the United States is up to billions of dollars [6]. According to previous reports, external and internal factors including environmental factors and susceptibility genes contribute to the pathogenesis of psoriasis [1, 2]. Additionally, it has been known that the interaction between immune cells and epidermal keratinocytes exerts a crucial function in the epidermal hyperplasia of psoriasis [7, 8]. Moreover, pro-inflammatory factors have been suggested to play key roles in the interaction between inflammatory cells and keratinocytes, which may be responsible for the occurrence, maintenance, and relapse of psoriasis [9–11]. Due to the lack of effective diagnosis methods for psoriasis, clinical diagnosis of psoriasis is largely dependent on Psoriasis Area and Severity Index (PASI) score [12]. To date, no effective cure strategy for psoriasis is available. Therefore, it is urgently required to explore novel biomarkers and pathways for new directions of psoriasis treatment.
MicroRNAs (miRNAs/miRs), as single-stranded and endogenous non-coding RNAs, play pivotal roles in multiple biological processes such as immunity, differentiation, inflammation, and proliferation [13–15]. MiRNAs negatively modulate their target genes by degrading mRNA and translational suppression via combining with their 3ʹ untranslated regions (UTRs) [16]. Previous documents have highlighted the functions of miRNAs in immune dysfunction [17], inflammatory response [18–20], and aberrant proliferation and differentiation of keratinocytes [21, 22], all of which promote the phenotypes of psoriasis. Recently, reports have revealed that the production of chemokines/cytokines regulated by the miRNAs/ targets network promotes the interaction between immune cells and epidermal keratinocytes [23, 24]. The above-mentioned reports show that miRNAs are promising therapeutic targets for the treatment of psoriasis. MiR-125a-3p is a novel miRNA that plays a significant role in the pathological processes of colorectal cancer, breast cancer, systemic lupus erythematosus, allergic rhinitis, and asthma [25–28]. Nonetheless, the functionality of miR-125a-3p in psoriasis remains completely unknown.
Toll-like receptor 4 (TLR4) is speculated to be a trigger of apoptosis, which also expresses a high level in peripheral blood mononuclear cells of psoriasis patients [29]. Moreover, accumulating evidence indicates the promotive role of TLR4 in psoriasis pathogenesis [30]. It has been documented that TLR4/NF-κB signalling is implicated in the modulation of inflammatory responses by miRNAs. For instance, miR-489-3p and miR-155 suppress the inflammatory response in psoriasis by repressing TLR4/NF-κB signalling [16, 31]. Through bioinformatic analysis, TLR4 was predicated as the downstream target of miR-125a-3p for its binding sites in both mice and human beings. Thus, we hypothesized that miR-125a-3p may regulate the TLR4/NF-κB pathway to play a role in psoriasis.
Aim
In this study, human keratinocytes (HaCaT cell line) and psoriatic mice were used to explore the role of miR-125a-3p and its underlying molecular mechanism by which miR-125a-3p modulates the inflammatory response in psoriasis.
Material and methods
Patients and specimen collection
Psoriasis patients (n = 35) and healthy controls (n = 35) were recruited from Wuhan No. 1 Hospital from June 2018 to May 2020. Patients with psoriasis enrolled in this study had not received topical therapy for 2 weeks or systemic therapy for at least 1 month prior to skin biopsy. Biopsies (6 mm) at the lesional site (Psoriasis) of psoriasis patients and the biopsies from healthy skin tissues (Normal) were collected. All participants signed written informed consent forms. This study was performed following the Declaration of Helsinki with the approval from the Ethics Committee.
Cell culture and treatment
HaCaT cells were purchased from Beijing YITA Biotechnology Co., Ltd (YT800227, Beijing, China). HEK293T cells were commercially obtained from Nanjing Cobioer Biosciences Co., Ltd (CBP60439, Cobioer, Nanjing, China). All these cells were cultured in DMEM (Gibco, Carlsbad, CA, USA) containing 10% foetal bovine serum (FBS; Gibco) in a humidified incubator with 5% CO2 at 37°C.
M5 was used to treat the cultured keratinocytes for inducing psoriasis-like alterations [32, 33]. Briefly, M5 cocktail containing recombinant human tumor necrosis factor-α (TNF-α; 10 ng/ml, #300-01 A, Peprotech), oncostatin M (10 ng/ml, #300-10, Peprotech), interleukin (IL)-22 (10 ng/ml, #200-22, Peprotech), IL-17A (10 ng/ml, #200-17, Peprotech), and IL-1α (10 ng/ml, #200-01 A, Peprotech) or equal volume of PBS as control was added to incubate HaCaT cells for 1 day.
Cell transfection
After digestion with trypsin, cells (5 × 105/well) were plated in 6-well plates. Upon reaching 60% confluence, cells were transfected with miR-125a-3p mimics (miR-125a-3p; 50 nM), miR-125a-3p mimics negative control (miR-NC; 50 nM), miR-125a-3p inhibitors (miR-125a-3p inhibitor; 50 nM), miR-125a-3p inhibitor negative control (NC inhibitor; 50 nM), empty pcDNA3.1 (Vector; 20 nM) or pcDNA3.1/TLR4 (TLR4; 20 nM) using the Lipofectamine™ 3000 (#L3000015, Invitrogen Inc., Carlsbad, CA, USA) for 1 day with 5% CO2 at 37°C as instructed by the manufacturer. The abovementioned oligonucleotides were purchased from Shanghai GenePharma Co., Ltd. Transfection efficiency was confirmed post transfection for 48 h via real-time quantitative polymerase chain reaction (RT-qPCR) analysis.
Cell Counting Kit-8 (CCK-8) assay
The transfected cells (5 × 103 cells/well) were plated in 96-well plates and incubated for 1 day. The percentage of cell proliferation was evaluated by adding ten microliters of CCK-8 (A311-01, Vazyme Biotech Co., Ltd., Nanjing, China) into each well and incubated for another 4 h at 37°C. Later, a microplate reader (Bio-Rad) was used to measure the optical density at 450 nm.
Imiquimod (IMQ)-induced psoriasis-like mouse model
Female C57BL/6N mice (21 ±2 g, 7 weeks old) were purchased from Beijing Vital River Laboratory Animal Technology Co., Ltd (Beijing, China). All animals were maintained in a room with controlled temperature (23 ±2°C), under 12-h light-dark cycles with free access to standard food and water. After acclimatization to the living environment for 1 week, the hair on back skin was shaved and the mice were treated with 5% IMQ cream (62.5 mg, Aldara, UK, n = 15) or vehicle cream (Vaseline, n = 15) at the same dosage daily from Day 1 to Day 5. Topical application of IMQ, a TLR7/8 ligand and potent immune activator, rapidly induces dermatitis closely resembling human psoriasis, critically dependent on the IL-23/IL-17 axis [34]. All experimental protocols were in line with the guidelines of the Animal Care and Use Committee of Wuhan No. 1 Hospital. The animal study was approved by the Laboratory Animal Welfare & Ethics Committee.
AgomiR treatment
Female C57BL/6N mice (n = 15/group) received intradermal injection of agomiR-125a-3p (5 nmol in 100 μl PBS) or indicated control (agomiR-NC), at Day -2 and Day -1 before the application of IMQ or Vaseline. AgomiR-125a-3p and corresponding control were synthesized by RiboBio Co., Ltd. (Guangzhou, China). PASI score, which was recorded daily throughout 5 consecutive days of IMQ treatment, was obtained by adding the scores of epidermal thickening, desquamation, and erythema of mouse skins in accordance with the clinical PASI score of psoriasis patients. The scoring criteria: 0, no damage, 1, mild, 2, moderate, 3, severe, and 4, extremely severe. Each item scores from 0 to 4, and the overall scores range from 0 to 12. At Day 6, mice were killed, and the skin tissues were excised. A part of the skin tissues was snap-frozen in liquid nitrogen for RNA/protein extraction and the remaining part was subjected to fixation with 4% paraformaldehyde for tissue embedding and sectioning.
Immunohistochemistry staining
Formalin-fixed tissue samples were embedded in paraffin and sliced into 5-μm thick sections. For hematoxylin and eosin (H&E) staining, after deparaffinization and rehydration, the sliced sections were stained with hematoxylin solution, followed by subsequent counterstaining in eosin-phloxine solution. The mounted slices were observed under a Nikon microscope (Nikon, Japan). As to Ki67 staining, the sliced sections were rehydrated and blocked with 3% H2O2, followed by incubation with primary antibodies against Ki67 (ab16667, 1 : 200 dilution, Abcam Inc., Cambridge, UK) for 12 h and HRP-labelled IgG (ab97080, 1 : 500, Abcam) for 2 h. After adding diaminobenzidine (DAB; Beyotime) chromogenic solution, a microscope was used to observe the sections and the percentage of Ki67 staining was calculated.
RT-qPCR analysis
Total RNAs from cells and tissues were extracted using TRIzol reagent (YT2188, YITA). Reverse transcription of cDNA from five hundred nanograms of RNA was performed using TaqMan cDNA synthesis kit (Thermo Fisher Scientific Inc., Reinach, Switzerland). Then, PCR was performed with SYBR Green Master Mix (Q131-02/03, Vazyme, Nanjing, China) on ABI 7500 FAST Real-Time PCR System (Applied Biosystems, Foster City, CA, USA). Sangon (Shanghai, China) synthesized the primers and the sequences of which were presented in Table 1. GAPDH (for mRNAs) or U6 (for miRNAs) functioned as endogenous reference. Relative RNA expression was calculated using the 2–ΔΔCt [35].
Table 1
Primer sequences used for RT-qPCR in this study
Western blot analysis
After lysing samples with RIPA lysis buffer (SY4680, YITA), the concentration of protein was determined using a Dxy_PIERCE BCA Protein Assay kit (23227, Thermo Scientific). Equal amounts of protein specimens (50 µg/g/lane) were resolved on 12.5% polyacrylamide gels at 130 V and then transferred to polyvinylidene difluoride membranes (Millipore, Billerica, MA, USA). The membranes were blocked with 2.5% non-fat milk at 37°C for 1 h and then probed with primary antibodies against TLR4 (HTA125, 1 : 1000, eBioscience, CA, USA), NF-κB p65 (ab207297, 1 : 1000, Abcam), p-NF-κB p65 (ab76302, 1 : 1000, Abcam), IL-1β (ab254360, 1 : 1000, Abcam), and GAPDH (ab181603, 1 : 10000, Abcam) in a 4°C refrigerator overnight with slow shaking. After rinsing with TBST three times, the membranes were incubated with secondary antibodies (ab288151, 1 : 10000, Abcam) at 37°C for 1 h. After washing the membranes again, the proteins were visualized using the ECL detection system (JMCDVISSP, Millipore) in accordance with the product manuals and quantified with ImageJ software v.1.52v (National Institutes of Health).
Luciferase reporter assay
The target genes of miR-125a-3p were predicated in TargetScan v.7.2 (http://www.targetscan.org/mamm_31/) database. TLR4-wild-type (Wt) and TLR4-mutant (Mut) generated by cloning the 3’UTR of TLR4 Wt and Mut plasmids into the pGL3 luciferase reporter vectors (Promega Corporation), respectively. HEK293T cells were co-transfected with TLR4 luciferase reporter constructs (1 µg), and miR-125a-3p (100 nM) or miR-NC (100 nM) using Lipofectamine 3000 at 37°C for 48 h. Later, luciferase activity was detected using a Dual-Luciferase Reporter Assay Kit system (Promega, Madison WI, USA) as per the manufacturer’s protocol.
Statistical analysis
All data are expressed as the mean ± standard deviation (SD) and analysed using SPSS 21.0 (IBM Corp. Armonk, NY, USA). Comparisons between two groups were analysed using Student’s t test. Differences among multiple groups were analysed using one-way analysis of variance (ANOVA) followed by Tukey’s post hoc tests. The threshold for significance was set at p-value less than 0.05. Each experiment was conducted at least three times.
Results
MiR-125a-3p is reduced in psoriatic-skin samples and M5-treated HaCaT keratinocytes
To investigate the role of miR-125a-3p in psoriasis, we examined its expression in psoriatic-skin samples and healthy donors. Psoriatic-skin samples showed significant downregulation of miR-125a-3p versus healthy skin tissues (Figure 1 A). In addition, miR-125a-3p expression in psoriatic samples was in negative correlation with PASI score of the corresponding patients (Figure 1 B). To probe into whether miR-125a-3p is dysregulated by psoriasis-associated inflammatory factors in vitro, in cultured keratinocytes, psoriasis-like inflammation was induced by treating HaCaT cells with M5 [32, 33], and miR-125a-3p expression was detected thereafter. As shown in Figures 1 C–J, higher expression of psoriasis-related genes (S100A7, S100A8, and S100A9) and inflammatory genes (CXCL1, CXCL2, IL-1β, CCL2, and CCL20) was observed in M5-treated HaCaT cells than in control cells. Interestingly, HaCaT cells treated with M5 exhibited significantly reduced expression of miR-125a-3p (Figure 1 K). The above findings showed that miR-125a-3p is poorly expressed in psoriasis skin tissues and M5-induced HaCaT cells.
Figure 1
Downregulation of miR-125a-3p in psoriasis patients and M5-treated keratinocytes. A – MiR-125a-3p expression in skin specimens from healthy donors (n = 35) and psoriasis patients (n = 35) by RT-qPCR analysis. B – Correlation of PASI score with miR-125a-3p expression in psoriatic biopsies of 35 psoriasis patients. C–J – Expression of psoriasis-related genes (S100A7, S100A8, and S100A9) and inflammatory genes (CXCL1, CXCL2, IL-1β, CCL2, and CCL20) in HaCaT cells by RT-qPCR. K – MiR-125a-3p expression in HaCaT cells with or without M5 treatment by RT-qPCR. Experimental data are expressed as the mean ± SD. **p < 0.01, ***p < 0.001
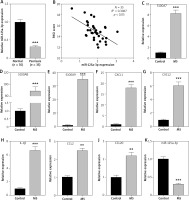
MiR-125a-3p suppresses the activity of keratinocytes in vitro
With the aim of exploring the function of miR-125a-3p in the pathogenesis of psoriasis, miR-125a-3p mimics and inhibitors were utilized to respectively elevate and downregulate miR-125a-3p expression. The miR-125a-3p mimics significantly enhanced, whereas miR-125a-3p inhibitors notably reduced, miR-125a-3p expression in HaCaT cells (Figure 2 A). As expected, cell proliferation was inhibited by miR-125a-3p mimics and was facilitated by miR-125a-3p inhibitors (Figure 2 B). Then, M5 was employed for inducing a psoriasis-like phenotype in HaCaT keratinocytes. As a result, the expression of psoriasis-related genes and inflammatory genes was increased after M5 induction. Meanwhile, the expression of these genes was suppressed by overexpression of miR-125a-3p in the absence or presence of M5 treatment (Figures 2 C–J). Furthermore, elevation of miR-125a-3p in HaCaT cells notably suppressed the levels of p-NF-κB p65 and IL-1β in the presence or absence of M5 induction (Figure 2 K). The miR-125a-3p inhibitors were adopted to suppress the expression of endogenous miR-125a-3p in HaCaT cells. As a result, the expression of S100A7, S100A8, S100A9, CXCL1, CXCL2, IL-1β, CCL2, and CCL20 along with p-NF-κB p65 and IL-1β was significantly upregulated upon miR-125a-3p knockdown with or without M5 treatment (Figures 2 L–T). Overall, miR-125a-3p represses the activity of keratinocytes in vitro by inhibiting cell proliferation and inflammation.
Figure 2
Effects of miR-125a-3p in vitro. A – Transfection efficiency of miR-125a-3p mimics or miR-125a-3p inhibitors in HaCaT cells. B – CCK-8 assay for assessing the proliferation of HaCaT cells transfected with miR-125a-3p mimics or miR-125a-3p inhibitors. C–J – The mRNA expression of psoriasis-related genes and inflammatory genes in HaCaT cells. K – The protein levels of p-NF-κB p65 and IL-1β in transfected HaCaT cells with or without M5 treatment by western blot analysis. L–S – The mRNA expression of psoriasis-related genes and inflammatory genes in HaCaT cells. T – The protein levels of p-NF-κB p65 and IL-1β in HaCaT cells. Experimental data are expressed as the mean ± SD. *p < 0.05, **p < 0.01, ***p < 0.001
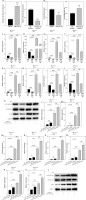
AgomiR-125a-3p attenuates the development of psoriasis induced by IMQ in vivo
To investigate the role of miR-125a-3p in vivo, agomiR-125a-3p was intradermally injected into the back of the shaved mice at Day -1, and Day 0. These mice were then treated with Vehicle/IMQ daily from Day 1 to Day 5, followed by sacrifice at Day 6 (Figure 3 A). The RT-qPCR results showed that miR-125a-3p displayed reduced expression in the IMQ + agomiR-NC group relative to the Vehicle group, while agomiR-125a-3p significantly elevated miR-125a-3p expression (Figure 3 B). Meanwhile, IMQ-induced high mouse PASI score was reduced by agomiR-125a-3p injection (Figure 3 C). Through H&E and Ki67 staining, it was found that the thickened and hyperplastic epidermis was caused by IMQ induction, and agomiR-125a-3p notably inhibited the hyperproliferative ability of keratinocytes (Figures 3 D–F). Additionally, IMQ treatment-induced upregulation of p-NF-κB p65 and IL-1β in mouse skins was significantly reversed upon agomiR-125a-3p injection (Figure 3 G). Moreover, IMQ-treated mice showed significantly increased expression of psoriasis-related genes and inflammatory genes, which was reversed by agomiR-125a-3p injection (Figures 3 H–N). All these data demonstrated that overexpressed miR-125a-3p suppresses psoriasis development in vivo via repressing hyperproliferation and inflammation of keratinocytes.
Figure 3
AgomiR-125a-3p attenuates IMQ-induced psoriasis in mouse models. A – Treatment regimens of agomiR-125a-3p in IMQ-induced psoriasis mice (n = 15/group). B – MiR-125a-3p expression in the groups of Vehicle, IMQ + agomiR-NC, and IMQ + agomiR-125a-3p. C – PASI score of mouse models in the indicated groups was recorded daily during the experimental periods. D – H&E staining for the histological feature of the back skin tissues. Scale bar: 100 μm. Ki67 staining for the proliferated cells. Scale bar: 50 μm. E, F – Skin epidermal thickness and the percentage of Ki67 staining were quantified. G – Protein expression of p-NF-κB p65 and IL-1β in each group. H–N – The expression of S100A7, S100A8, S100A9, CXCL1, CXCL2, IL-1β, CCL2, and CCL20 genes in back skin tissues from mouse models. N = 15/group. Experimental data are expressed as the mean ± SD. **p < 0.01, ***p < 0.001
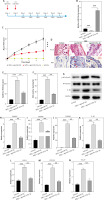
MiR-125a-3p targets TLR4
TargetScan was employed to predict the putative targets of miR-125a-3p. TLR4, which has been shown to promote psoriasis development by targeting NF-κB signalling pathway [30, 36], was predicted as a target of miR-125a-3p. Meanwhile, predicated consequential pairing of TLR4 and miR-125a-3p in both human and mouse were shown by this database (Figure 4 A). To validate the combination between TLR4 and miR-125a-3p, a luciferase reporter construct which harboured the WT 3°C UTR of TLR4 was generated. A mutated version of TLR4 3’-UTR was also generated in which seven complementary nucleotides UCACCUG in the binding site were altered to AGUGGAC (Figure 4 B). After co-transfecting miR-125a-3p mimics or miR-NC with TLR4-Wt or TLR4-Mut into HEK293T cells, miR-125a-3p mimics notably suppressed the luciferase activity of TLR4-Wt, but this suppression was lost when con-transfection with TLR4-Mut occurred (Figure 4 C). Of note, TLR4 protein expression was inhibited by miR-125a-3p mimics whereas was enhanced by miR-125a-3p inhibitors in HaCaT cells (Figure 4 D). Moreover, TLR4 expression levels were significantly elevated in M5-treated HaCaT cells and psoriasis lesional skins (Figures 4 E–F). In addition, TLR4 protein bands were significantly weakened in the IMQ + agomiR-125a-3p group compared to those from the IMQ + agomiR-NC group (Figure 4 G). More importantly, a positive correlation between TLR4 expression and PASI score as well as a negative correlation between miR-125a-3p expression and TLR4 protein positivity was identified in both psoriasis skin tissues and IMQ-induced mouse models (Figures 4 H–K). Taken together, TLR4 is a direct downstream target of miR-125a-3p.
Figure 4
MiR-125a-3p targets TLR4. A – Predicated consequential pairing of TLR4 and miR-125a-3p shown by TargetScan database. B – Constructed TLR4-Mut and the predicated binding site between TLR4 and miR-125a-3p. C – Luciferase reporter assay for validating the combination between miR-125a-3p and TLR4. D, E – The protein expression of TLR4 in the transfected- or treated-HaCaT cells. F – TLR4 mRNA expression in skin tissues from healthy donors (n = 35) and psoriasis patients (n = 35) by RT-qPCR analysis. G – Protein expression of TLR4 in mice. H, I – Correlation of TLR4 protein positivity and PASI scores in psoriasis patients (n = 35) or IMQ-stimulated psoriasis mice (n = 35). J, K – Correlation of TLR4 protein positivity and miR-125a-3p in psoriasis patients (n = 35) or IMQ-stimulated psoriasis mice (n = 35). Experimental data are expressed as the mean ± SD. ***p < 0.001
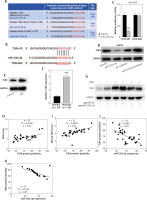
MiR-125a-3p inhibits the proliferation and inflammation in HaCaT cells by suppressing TLR4
Rescue experiments were performed to further reveal the role of the miR-125a-3p/TLR4 axis in HaCaT cells. Briefly, pcDNA3.1/TLR4 was transfected into HaCaT cells to overexpress TLR4 expression (Figure 5 A). MiR-125a-3p mimics (or miR-NC) were transfected with pcDNA3.1/TLR4 in HaCaT cells and then RT-qPCR and western blot analysis were conducted. We found that TLR4 upregulation eliminated the suppressive influences of miR-125a-3p mimics on cell proliferation, inflammatory factors, and inflammatory pathways in HaCaT cells (Figures 5 B–K). Our study revealed that miR-125a-3p represses keratinocyte activity by directly reducing TLR4 expression.
Figure 5
Overexpressed TLR4 reverses the influences of miR-125a-3p on HaCaT cells. A – Overexpression efficiency of TLR4 in HaCaT cells by RT-qPCR. B – Cell proliferation in the miR-NC, miR-125a-3p, and miR-125a-3p + TLR4 groups by CCK-8 assay. C–J – Gene mRNA expression in the indicated groups. K – Protein levels of p-NF-κB p65 and IL-1β in the indicated groups. Experimental data are expressed as the mean ± SD. *p < 0.05, **p < 0.01, ***p < 0.001
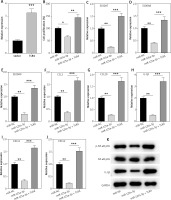
Discussion
Psoriasis is believed to be a result of the impaired cross-talk between immune cells and keratinocytes caused by environmental and genetic factors [9]. MiRNAs are evolutionarily conserved RNAs which mainly post-transcriptionally regulate gene expression [13, 15]. Recently, the dysregulated miRNAs have been disclosed in the development of psoriasis, suggesting their crucial role and potential diagnostic function in this disease [37, 38]. The focus of current research is the study of miRNAs in the dysregulation of immune cells as well as the abnormality of differentiation and/or proliferation of keratinocytes [39]. Here, miR-125a-3p was poorly expressed in the lesional skin of psoriasis patients, M5-treated HaCaT cells, and IMQ-treated psoriasis-like mice. Functional experiments revealed that miR-125a-3p mimics repressed the proliferation and inflammation of HaCaT cells in vitro while agomiR-125a-3p attenuated IMQ-induced psoriasis mice in vivo. Moreover, TLR4, which was targeted by miR-125a-3p, reversed the effects of miR-125a-3p on HaCaT cells.
Accumulating literature illuminates that PASI is a crucial indicator in the assessment of clinical psoriasis. Some studies also demonstrate that the expression of miRNAs in psoriasis patients is in negative correlation with the PASI score [40]. In our investigation, miR-125a-3p expression was also negatively correlated with PASI in psoriasis patients, revealing that miR-125a-3p might be related to the severity of psoriasis. The hyperproliferation of keratinocytes is a pivotal histopathology of psoriasis [41]. An emerged hotspot to date is the modulation of keratinocytes via psoriatic-related miRNAs. For example, miR-125b is poorly expressed in psoriasis patients and its elevation results in the inhibited differentiation and proliferation of keratinocytes [42]. Suppression of miR-145-5p promotes keratinocyte hyperproliferation and skin inflammation in psoriasis [23]. However, the correlation between miR-125a-3p and psoriasis remains completely unknown, and the focus of miR-125a-3p recently is its role in cancer development such as colorectal cancer and breast cancer [25, 26]. Notably, miR-125a-3p shows downregulated expression in kidney tissues of systemic lupus erythematosus mice. In the same study, overexpression of miR-125a-3p causes repression of fibrosis in experimental mice [27]. Consistently, miR-125a-3p expression in our study was not only reduced in psoriasis patients, but also suppressed in M5-treated HaCaT cells, and IMQ-stimulated psoriasis-like mice. Moreover, miR-125a-3p elevation inhibited the excessive proliferation of keratinocytes both in vitro and in vivo as manifested by the inhibited proliferation of M5-treated HaCaT cells and the reduced epidermal thickness and Ki67 positive cells in IMQ-generated psoriasis-like mice. On the contrary, knockdown of miR-125a-3p contributed to the enhanced proliferative ability of HaCaT cells.
Suppression of inflammatory response might become an effective strategy for treating psoriasis. A host of studies reveal the critical role of the immune system whereby numerous inflammatory cytokines generated by immune cells promote the proliferation of keratinocytes [43]. NF-κB is a crucial transcription factor in psoriasis [44] translocating from the cytoplasm to the nucleus upon stimulation and inducing gene expression to mediate innate and acquired immune modulation, anti-apoptotic stimuli, inflammatory response, and cellular adhesion [45, 46]. IL-1β, IL-4, IL-22, interferon-γ (IFN-γ), and TNF-α activated by NF-κB serve central roles in inflammation [47]. In the present investigation, p-NF-κB p65 and IL-1β were all suppressed by overexpressed miR-125a-3p or enhanced by silenced miR-125a-3p in HaCaT cells with or without M5 treatment. In vivo study revealed that agomiR-125a-3p inhibited the levels of p-NF-κB p65 and IL-1β in IMQ-stimulated psoriasis skin tissues. Additionally, the expression of psoriasis-related genes (S100A7, S100A8, and S100A9) and inflammatory genes (CXCL1, CXCL2, IL-1β, CCL2, and CCL20) in M5-treated HaCaT cells was reduced by upregulated miR-125a-3p whereas increased after transfection of miR-125a-3p inhibitors. The anti-inflammatory effect of miR-125a-3p in this study was consistent with the previous study in rheumatoid arthritis and diabetic cardiomyopathy [48, 49].
It is well-known that miRNAs negatively modulate their downstream genes by translational suppression and mRNA degradation [16]. Combined with bioinformatics prediction and luciferase reporter assay, TLR4 was confirmed as the target of miR-125a-3p. TLRs are important mediators of adaptive and innate immunity which can be activated by pro-inflammatory cytokines upon stimuli thereby activating the inflammatory response [50]. The correlation between chronic plaque psoriasis and intronic variant of TLR4 has been uncovered by researchers [51]. Additionally, depletion of TLR4 mitigates psoriasis-like inflammation in animal models [52]. In our current study, TLR4, displaying a high expression level in the skin tissues of psoriasis patients, was reduced by miR-125a-3p mimics but increased by miR-125a-3p inhibitors in HaCaT cells. Additionally, IMQ-induced mice also exhibited downregulated expression of TLR4 after agomiR-125a-3p injection. Rescue experiments demonstrated that miR-125a-3p mimics-suppressed proliferation and inflammatory response in HaCaT cells were reversed by elevated TLR4. Interestingly, TLR4 signalling is also implicated in the activation of NF-κB during the immune response and silenced TLR4 represses the activation of NF-κB signalling pathway [53]. Our study validated that the reduced protein levels of p-NF-κB p65 and IL-1β caused by miR-125a-3p upregulation was enhanced after pcDNA3.1/TLR4 transfection.
Conclusions
Conclusively, our study first reported that miR-125a-3p ameliorated hyperproliferation of keratinocytes and psoriasis-like inflammation by targeting TLR4-mediated NF-κB signalling pathway. However, several limitations still existed in our study. First, due to the complexity of molecular regulatory mechanisms, other downstream targets of miR-125a-3p deserve further exploration. Second, given the crucial interaction between epidermal keratinocytes and immune cells in the epidermal hyperplasia of psoriasis, the impact of miR-125a-3p on immune cells also requires investigation in the future.